Sox2 guides R-loops to drive cellular reprogramming to pluripotency
Abstract
Discussion
R-loops are structures consisting of an RNA-DNA duplex and an unpaired strand of DNA and are formed when nascent RNAs exit the RNA polymerase channel and hybridize with homologous genomic loci present on the DNA strand that causes the double helix to open generating an ssDNA.
Yaoyi et al. in this study [1] wanted to investigate the regulatory mechanisms of R-loops in the cellular reprogramming process: the process that allows the dedifferentiation of terminally differentiated cells into pluripotent stem cells. They used an in vitro model, the mouse embryonic fibroblasts (MEFs), and treated them with Yamanaka cocktail that contained 4 transcription factors (Oct4, Sox2, Klf4 and c-Myc). With this cocktail, the MEF cells were reprogrammed into pluripotent stem cells (iPSCs). The efficiency of reprogramming was measured by using an endogenous Oct4-GFP gene that marks properly reprogrammed cells.
The process was observed for 7 days, and cells were collected and subjected to RNA-seq, to analyze the gene expression, and ssDRIP-seq [2], to study the R-loop dynamic (Fig. 1A). The last one is a technique that involves genomic DNA extraction and fragmentation in which the fragments are incubated with the S9.6 antibody (specific for the R-loop structures), followed by immunoprecipitation and sequencing.
The authors found that the R-loops change over the cell reprogramming process. This suggests that these structures may be crucial in this process. RNA-seq analysis showed that there were expression changes during this process detected in the same days as ssDRIP-seq, suggesting a correlation between gene expression and the dynamics of the R-loops, so the authors compared the data of the two analyses. It was seen that the dynamics of the R-loops changed earlier in the observed time than the change in gene expression. From this it can therefore be suggested that the R-loops influence gene expression during reprogramming.
To better understand how R-loops work in the reprogramming process, Yaoyi L. and his collaborators took in consideration the Ribonuclease H1 (RNaseH1) [3]. It is one of the enzymes that regulate the homeostasis of R-loops by cutting and splitting them. The authors manipulated the activity of this enzyme by employing RNaseH1 knockdown and mutants.
The RNaseH1 knockdown is an experimental technique through which the gene expression is reduced; it was performed by using two independent short hairpin RNAs (shRNAs). RNAseqH1 knockdown decreased the number of GFP positive iPSCs, thus affecting the cell reprogramming.
Further, the authors employed three different mutants: 1) mutation of the evolutionarily conserved catalytic domain (D209N), 2) mutation of multiple residues of the binding domain, 3) mutation of both the binding domain and the catalytic site. Only the mutant (RNaseH1 D209N) abolished the catalytic activity of RNaseH1. They also noticed that an overexpression of RNaseH1D209N significantly inhibited the formation of GFP+ colonies compared to the control group or overexpression of the WT RNaseH1. Altogether, these data indicated that both the overexpression of RNaseH1D209N and the knockdown of RNaseH1 impaired the reprogramming efficiency.
This study also shows a way to recover the reprogramming process, inactivated by the inhibition of RnaseH1. The authors thought that one of the four transcriptional factors (Yamanaka cocktail) may have a recovery effect to this process. They performed reprogramming experiments with the knockdown or the overexpressed RNaseH1D209N together with Oct4 – Sox2 – Klf4 – c-Myc in different combinations. From these data the authors discovered that only Sox2 has a role in the recovery of reprogramming. In fact, they noticed that an increase of Sox2 amount induced an increase of iPSCs colonies while there is no dose-dependent effect for the other three factors.
Since Sox2 has this ability, they performed ssDRIP-seq and RNA-seq cells treated with only Oct4, Klf4 and c-Myc discovering that Sox2 influence R-loops dynamic and gene expression during reprogramming.
The results obtained indicate that the R-loops could be upregulated both by overexpressing RNaseH1D209N and overexpressing Sox2. However, R-loops induced by RNaseH1D209N have a detrimental effect on reprogramming, while Sox2-mediated R-loops facilitate reprogramming. They then hypothesized and demonstrated that RNaseH1D209N and Sox2 could regulate different R-loops, so while RNaseH1D209N increases the accumulation of aberrant R-loops, Sox2 increases the accumulation of R-loops “benign” for reprogramming process (Fig. 1B).
How does Sox2 works to restore de-differentiation? Regulates R-loops directly or indirectly?
To answer this question the authors performed an Electrophoretic Mobility Displacement Assay (EMSA), a system to identify and characterize protein-nucleic acid interactions, and showed that Sox2 could not bind directly to the R-loops. So, probably, Sox2 could regulate the R-loop by interacting with other factors. To identify any protein cofactors associated with the R-loops they performed an immunoprecipitation analysis with the R-loop specific antibody. They find out Ddx5 cofactor that binds R-loops and also Sox2.
Previous data [4] indicated that Ddx5 acts as a barrier to reprogramming, through the repression of Rybp (key protein for pluripotency and differentiation), maintaining the activation of somatic genes and silences the expression of pluripotent genes. The authors investigated the Sox2-Ddx5 interaction discovering that Sox2 can inhibit Ddx5 activity (Fig. 1C).
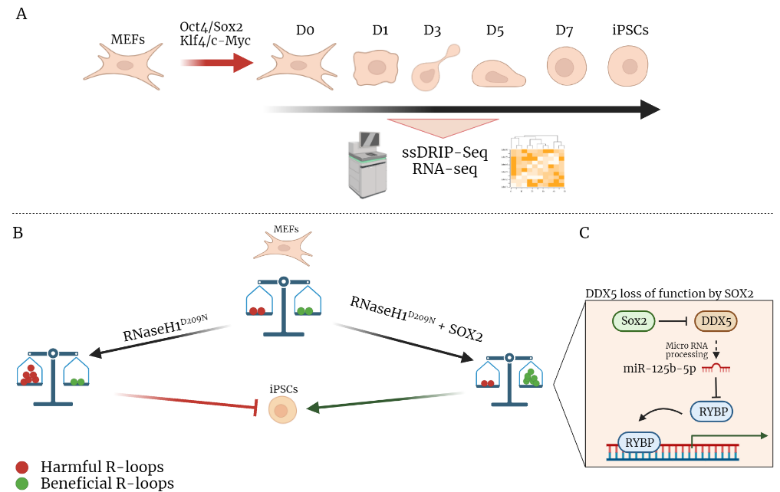
Fig 1. A) Reprogramming process followed in 7 days and analyzed with ssDRIP-se and RNA-seq. B) MEFs treated with RNaseH1D209N and Sox2 with the two different results. C) Focus on the Sox2 mechanism of reprogramming process recovery allowing the expression of pluripotential genes.
Conclusions
In conclusion, this article proved that some R-loops were harmful so their aberrant accumulation could inhibit reprogramming, while another fraction of R-loop was beneficial. The homeostasis of these R-loops is essential for reprogramming and an unbalanced of them inhibited this process. A proof of the R-loops role in the reprogramming process is obtained through the manipulation of RNaseH1 causing an accumulation of aberrant R-loops and the inhibition of reprogramming. The authors find out that the well know role of Sox2 in reprogramming is at least partially related to the maintenance of R-loops.
This article is well structured and explained in a clear way, but researchers have not clarified why the mutation of both the binding domain and the catalytic site do not inhibit the reprogramming process. They mentioned to the fact that the absence of the binding domain means that the enzyme does not bind to the R-loop structures and does not protect them from further enzymes that could cut them. Therefore, it does not cause the aberrant accumulation, as it happens instead with the modification of the catalytic domain only. In our opinion, further studies are required to better investigate the action mechanism of RNaseH1, investigating the probable presence of other factors that play a role in the homeostasis of R-loops.
References
- Li Y, Song Y, Xu W, Li Q, Wang X, Li K, Wang J, Liu Z, Velychko S, Ye R, Xia Q, Wang L, Guo R, Dong X, Zheng Z, Dai Y, Li H, Yao M, Xue Y, Schöler HR, Sun Q, Yao H. R-loops coordinate with SOX2 in regulating reprogramming to pluripotency. Sci Adv. 10;6 (2020);
- Xu W., Xu H., Li K., Fan Y., Liu Y., Yang X., Sun Q. The R-loop is a common chromatin feature of the arabidopsis genome. Nat. Plants 3, 704–714 (2017);
- L. Wahba, J. D. Amon, D. Koshland, M. Vuica-Ross. RNase H and multiple RNA biogenesis factors cooperate to prevent RNA: DNA hybrids from generating genome instability. Mol. Cell 44, 978–988 (2011);
- H. Li, P. Lai, J. Jia, Y. Song, Q. Xia, K. Huang, N. He, W. Ping, J. Chen, Z. Yang, J. Li, M. Yao, X. Dong, J. Zhao, C. Hou, M. A. Esteban, S. Gao, D. Pei, A. P. Hutchins, H. Yao, RNA helicase DDX5 inhibits reprogramming to pluripotency by miRNA-based repression of RYBP and its PRC1-dependent and -independent functions. Cell Stem Cell 20, 462–477.e6 (2017).