Stem cells can trim their telomeres – and for good reasons too: here is how and why they do it
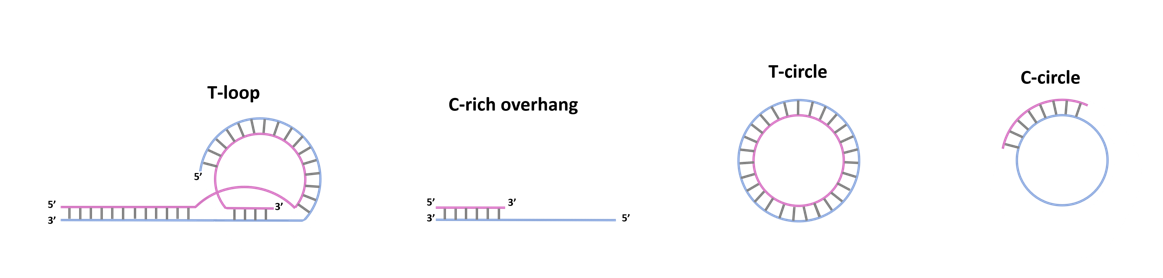
In 2009, biologists Elizabeth Blackburn, Jack William Szostak, and Carol Greider were awarded the Nobel Prize in Physiology or Medicine for their researches on “the discovery of how chromosomes are protected by telomeres and the enzyme telomerase” [1]. The structures that were discovered, telomeres, are located at the ends of eukaryotic chromosomes; they are composed of the repeated DNA sequence 5’-TTAGGG-3’ and the complementary sequence of the opposite hemielix [2]. At the extremity of the telomere, the 5’-3’ filament bends towards itself, binding a downstream portion of the 3’-5’ filament, thus forming what is known as “T-loop” [3]. The discovery of telomeres was so groundbreaking, due to the fact that their maintenance proved to be crucial in preserving staminality in stem cells: the very ends of the DNA get shortened at each division cycle, finally leading to cell senescence or apoptosis [4]. Stem cells are able to evade this fate by elongating their telomeres through the activation of an enzyme called telomerase, counteracting the shortening effect of cell division.
Another mechanism some cells use to elongate their telomeres is the so-called “alternative lengthening of telomeres” (ALT). ALT is supposed to act via homologous recombination between telomeres, shortening one chromosome while lengthening its sister [5]. Till this date, research has been mainly focused on how telomeres which are too short can damage stem cells, as well as on the tools these cells use in order to elongate them. A recent study by Rivera et al., published in Nature Structural & Molecular Biology [6], takes the courage to explore the opposite scenario: which problems arise when telomeres are too long and how do stem cells cope with them?
To test whether telomeres could cause noxious effects when exceeding a certain length, researchers started by overextending the telomeres of human embryonic stem cells (hESCs). When modified in this way, the cells showed higher frequencies of metaphase chromosomes with multitelomeric signals (MTS), a marker indicating replication defects. A significant increase in telomere dysfunction-induced foci (TIFs), a marker indicating telomere fragility, was also observed. The data suggest that excessive telomere length in hESCs induces accumulation of telomeric DNA replication defects, consequently producing more fragile telomeres. Telomeres seem therefore to have an optimum length: let them shorten too much and senescence or apoptosis will occur, make them too long and you will generate flimsy ends. Having stated that a homeostasis regulating telomere length must exist in order for stem cells to thrive, it remained obscure what mechanisms the cell adopts to achieve it. It looks like just half of the story was clear: for sure, telomerase and ALT could be used to elongate short telomeres, but how one reversed excessive elongation was still unknown.
Eager to shed light on this blind spot, researchers took a counterintuitive approach: they started by looking for ALT’s markers in stem cells. Cells that do have ALT activity, are known to show five specific hallmarks: high concentrations of 5’ C-rich telomeric overhangs (C-rich overhangs), T-circles and C-circles (Figure 1) , as well as high incidence of telomere sister chromatide exchange events (t-SCEs) and the presence of ALT-associated promyelocytic leukemia bodies (APBs) containing telomeric chromatin.
hESCs and human induced pluripotent stem cells (hiPSCs) appeared to have the first three markers, but lacked the latter two. If the cells performed ALT, all markers should have been visible; given the data obtained, that was clearly not the case. Nonetheless, C-rich overhangs, T-circles and C-circles were still detected: if ALT was not occurring, what was causing the production of these markers?
We shall now remind the reader that all these three markers are made of telomeric sequences: therefore, it might not seem unsound to hypothesize them to be the “leftovers” of supposed telomere trimming events. Such events would presumably occur when telomeres are too long, in order to restore their ideal length and avoid telomere fragility. If all these speculations were true, artificially elongating the telomeres in hESCs would imply an increasing accumulation of the three markers. Elongating telomeres, researchers detected higher concentrations of T and C circles. C-rich overhang concentrations remained unchanged, though: the reason was, supposedly, that degradation led by endogenous esonucleases restored the original C-rich overhang concentration. As it has been shown, the data supported the hypothesis, but still, it was clearly not enough to prove it with a good level of confidence: it was time to investigate deeper how the markers formed.
When hESCs were treated with RHSP4, which interferes with telomere replication, an accumulation of C-circles was measured. The concentration of C-circles got also higher when the proteins DNA2 and SMARCAL1, which restore replication defects, were silenced by siRNA. This data suggests C-circles could be extruded from stalled replication forks to restore telomeric DNA replication. Longer telomeres have already been shown to bear more replication defects: this mechanism would allow the reversal of these defects, while at the same time reducing the excessive telomere length that caused them in the first place. The origin of the remaining markers was analyzed by similar means; XRCC3 and Nbs1 were silenced one at a time, causing the accumulation of, respectively, C-rich overhangs and T-loops. This implies, the two proteins are responsible for C-rich overhangs and T-circles formation. It was anyways when both XRCC3 and Nbs1 were simultaneously silenced that something truly remarkable occurred: telomeres started to get longer. This result can be easily explained as follows: XRCC3 and Nbs1 cause the resolution of T-loops in T-circles and C-rich overhangs, reducing in this way telomere length when that exceeds a certain point. Silencing of XRCC3 and Nbs1 will translate in telomere trimming inhibition, allowing it to increase in length.
Nobel laureate Elizabeth Blackburn, mentioned at the beginning of this article, once stated: “Telomeres are buffers. They are like the tips of shoelaces. If you lose the tips, the ends start fraying” [7]. It is perhaps time to update that common view: just like when being too short, overly long telomeres will also cause serious issues; stem cells have evolved to cope with that and now we know more about how they do it.
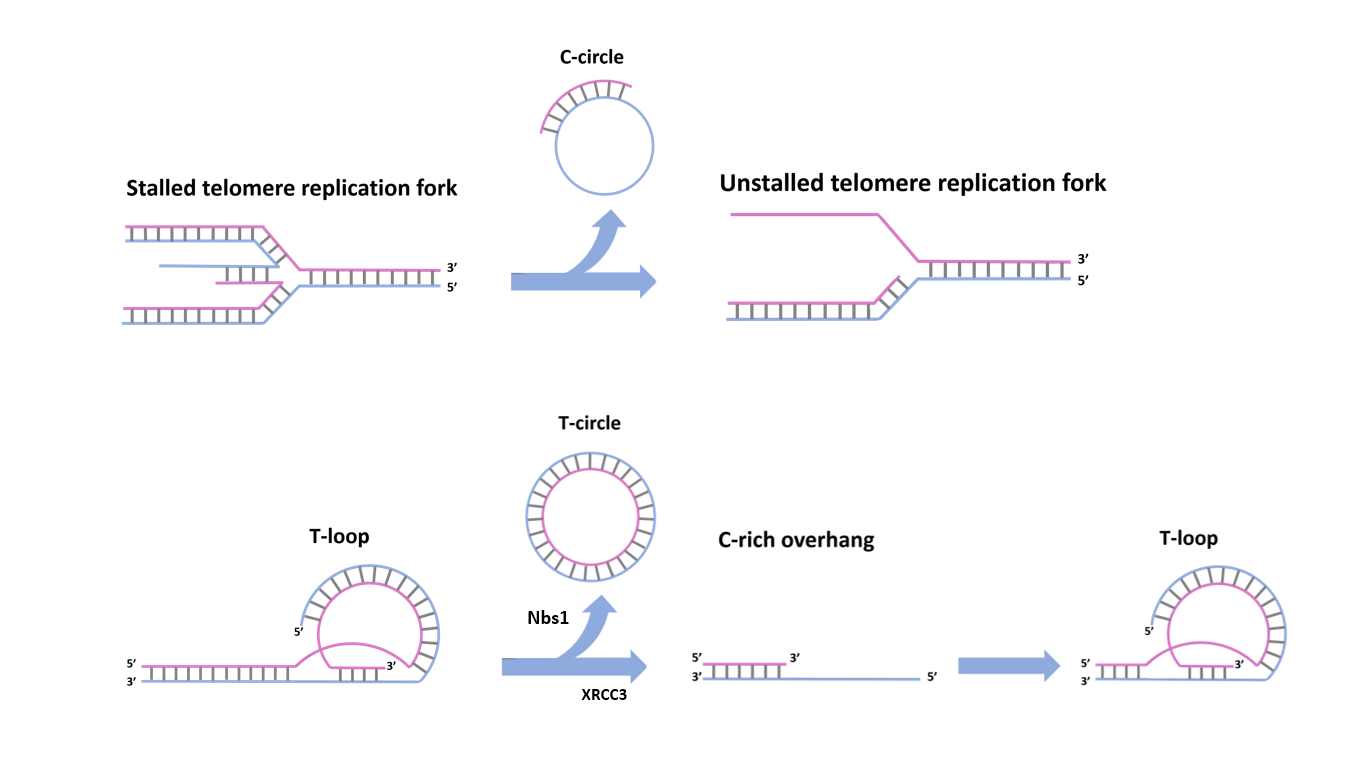
Fig. 1 – Telomere trimming, mediated by Nbs1 and XRCC3
References
- https://www.nobelprize.org/prizes/medicine/2009/summary/
- Makarov, V.L., Hirose, Y. & Langmore, J.P. Long G tails at both ends of human chromosomes suggest a C strand degradation mechanism for telomere shortening. Cell 88, 657–666 (1997).
- Griffith, J.D. et al. Mammalian telomeres end in a large duplex loop. Cell 97, 503–514 (1999).
- Olovnikov, A.M. A theory of marginotomy. The incomplete copying of template margin in enzymic synthesis of polynucleotides and biological significance of the phenomenon. J. Theor. Biol. 41, 181–190 (1973).
- Bryan, T.M., Englezou, A., Dalla-Pozza, L., Dunham, M.A. & Reddel, R.R. Evidence for an alternative mechanism for maintaining telomere length in human tumors and tumor-derived cell lines. Nat. Med. 3, 1271–1274 (1997).
- Rivera, T., Haggblom, C., Cosconati, S., Karlseder. A balance between elongation and trimming regulates telomere stability in stem cells Nat. Struct. Mol. Biol. 24, 30–39 (2017)
- https://www.nytimes.com/2007/07/03/science/03conv.html