The lone virus dies but the pack survives
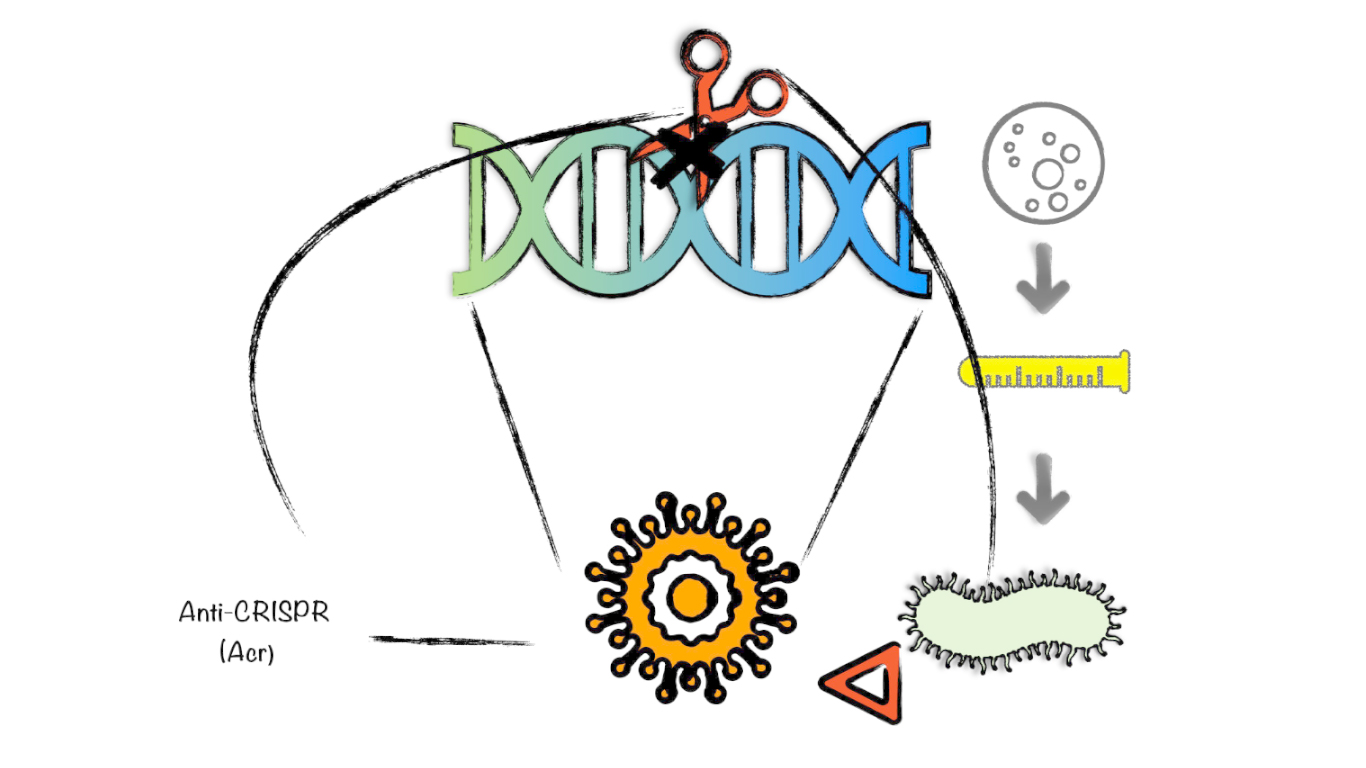
The battle for survival between bacteria and phages does not end. To survive some smart phages have evolved anti-CRISPR enzymes that allows them to shut down the CRISPR-based immunity and support biotechnological applications for which the purpose is inactivate Cas12a.
The bacteria use CRISPR- Cas system as immune defense in response of viral infection. CRISPR-Cas immunity generally occurs through three steps: adaptation, expression, and interference. During the first one, fragments of invading foreign DNA – protospacers – are integrated into the CRISPR (Clustered Regularly Interspaced Short Palindromic Repeats) array. While expression, the CRISPR array is transcribed and processed to yield single repeat-spacer RNA known as CRISPR- RNA (crRNA). crRNAs are subsequently complexed with one or more Cas (CRISPR- associated) proteins to form ribonucleoprotein surveillance complexes. During interference, these complexes search foreign nucleic acid through complementarity with the crRNAs and cut it.
Before the discovery of anti-CRISPR proteins, the acquisition of point mutations within the protospacer or the PAM (protospacer adjacent motif) or seed sequence, a region of the protospacer that must have perfect complementarity to the crRNA, have been shown to be enough for phages to escape CRISPR-Cas immunity. Since CRISPR-Cas system is adaptive and can re-acquire new spacers, it seemed that bacteria have won. In 2013, the first active counter-mechanism to CRISPR-Cas immunity, anti-CRISPR proteins, was identified within a small group of closely relate Pseudomonas aeruginosa phages. To date, have been classified a total of 40 distinct families of anti-CRISPR proteins. The peculiarity of Acr proteins is they share no common sequence or motif among them. The great diversity is both structural and functional. Anti-CRISPRs may be able to prevent Cas proteins from acquiring new spacers. They could potentially also block transcription of the CRISPR array or processing of the transcript into mature crRNAs. The anti-CRISPR proteins that inhibit the interference bind directly to the effector protein complex and could thereby inhibit one of three steps: crRNA loading, target DNA binding, or target DNA cleavage. Anti-CRISPRs that obstruct crRNA loading have not yet been reported, but anti-CRISPRs that block both target DNA binding and cleavage have [1].
The discovery of type V-A anti-CRISPR proteins was an exciting advance, as Cas12a, the effector protein in type V-A CRISPR-Cas systems, is an attractive alternative to Cas9 for genome editing purposes. A new study recently published in Nature Struct. Mol. Biol by Knott and collaborators [2] shows that AcrVA1 uses enzymatic activities previously unobserved to inactivate CRISPR-Cas12a system. The formation of the Cas12a-crRNA complex is essential for targeting and cutting viral DNA. The nuclease Cas12a catalyzes both single-turnover target DNA cutting (cis-cleavage) and multiple-turnover non-target ssDNA cutting (trans-cleavage). After the cis-cleavage, the PAM-proximal DNA remains bound to the Cas12a-crRNA complex: this keep Cas12a active which permits unusual trans cleavage. Knott and collaborators wondered in the first instance whether AcrVA1, AcrVA4, AcrVA5 could prevent the formation of the complex, then what their effect was once the complex was already formed (strategy that may have evolved to disable an activated and trans-cleaving Cas12a). The results showed that AcrVA1, although it is not a RNAse, cuts the guide crRNA; the cleaved guide fragment and AcrVA1 are released, the inhibitor then can turn over and cleave crRNA in other copies of the Cas12a effector complex (Fig. 1a). The shortened crRNA still bound to Cas12a is not competent for binding target DNA, as it lacks complementarity to the target region of the dsDNA. In addition to that, the limited length of the crRNA could prevent the formation of a hybrid structure stable. AcrVA1 triggers endoribonucleolytic truncation of both mature and pre-crRNA in the presence of three Cas12a orthologs (Moraxella bovoculi, Acidaminococcus sp., Lachnospiracea bacterium); it alone lacks RNA-cleavage activity, but it robustly cleaves the crRNA within the effector complex. At sub-stoichiometric concentrations of AcrVA1, crRNA cleavage is still observed at maximal levels. AcrVA1 activity is multiple turnover where cleavage of a crRNA will inactivate Cas12a–crRNA complexes [3]. Neither residues in the Cas12a active site nor metal ions (MgCl2 or EDTA) are required for the AcrVA1-dependent truncation of crRNA, although the exact mechanism of AcrVA1’s activity remains unclear. At present, is know that the activity of AcrVA1 is independent of spacer’s sequence or length. The authors did not perform experiments to figure out whether AcrVA1 recognizes a specific site of crRNA. Notably, AcrVA1 has previously been shown to potently inhibit a broad range of Cas12a orthologs suggesting that this activation can occur across several Cas effector sequences [4]. The prowess of AcrVA1 to inhibit all three Cas12a orthologs used, proposes that it might exploit an evolutionary conserved domain of Cas12a. In fact, the PAM-interacting domain is severely required for process of inhibition.
AcrVA4 presents a strategy that has also been described for AcrIIC3, which targets NmeCas9 (Neisseria meningitidis): it blocks the DNA binding forming a dimeric complex composed with the ribonucleoprotein. The results indicate that two dimers (AcrVA4-ribonucleoprotein) interact with each other to form a symmetrical complex (Fig. 1b). In this case, is required 1:1 stoichiometry between AcrVA4 and Cas effector: this highlights the peculiarity and the power of AcrVA1 compared to AcrVA4.
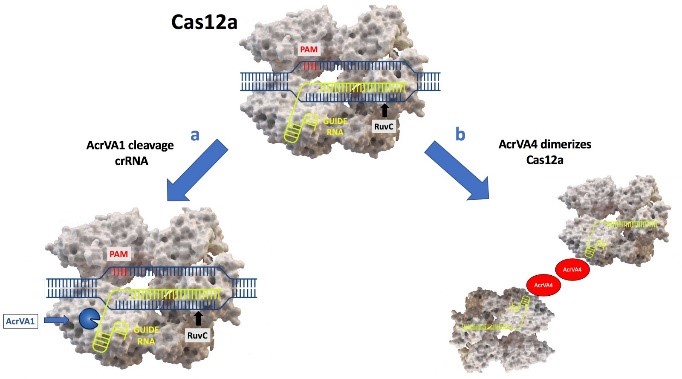
Fig. 1 | a) AcrVA1 cleaves the crRNA bound to Cas12a. b) AcrVA4 dimerizes Cas12a.
At high concentrations, AcrVA4 triggers the release of dsDNA bound to dLbCas12a. AcrVA4 has no effect on DNA bound to Cas12a wild type: Knott et al. assume that the release of the PAM-distal dsDNA fragment might prevent AcrVA4 displacing the PAM-proximal dsDNA bound to guide crRNA. As regard AcrVA5, are given only few information. By contrast, a recent paper [5] indicates that it’s an acetyltransferase that modifies lysine residues involved in ‘reading out’ the PAM. Both AcrVA1 and AcrVA5 present a multi-turnover kinetics. The versatility of these inhibitors reflected their efficiency: they work on several levels. If the complex Cas12acrRNA-DNA target has not yet formed AcrVA1 prevents DNA binding, but if the complex was already formed AcrVA4 can dislodge dsDNA bound to dCas12a.
Perhaps, the Acr enzymes are uncommon as a result of the complex set of requirements for the evolution of enzymatic activities. It is still unclear whether the Acr enzymes are more effective than Acr proteins. These last could provide a long-term inhibition by remaining bound to the Cas effector, while AcrVA1 or AcrVA5 may provide only temporary inactivation of Cas12a. Recent studies have suggested that Cas12a can efficiently replace its guide to reset the complex which could reduce the efficacy of the truncation of crRNA by AcrVA1 [6]. One obvious way bacteria evade anti-CRISPR inhibition is to harbor multiple types of CRISPR-Cas systems, as seen in Pseudomonas and Moraxella species. By having several active CRISPR-Cas systems, bacteria can protect themselves from phages that have anti-CRISPRs for a single type of CRISPR-Cas system. However, phages may also respond to this evolutionary pressure. For example, anti-CRISPR protein AcrIF6 has dual specificity and is able to inhibit both type I-E and I-F CRISPR-Cas systems [7]. Another way is by acquiring mutations in the Cas proteins so that they escape targeting by the anti-CRISPR proteins. Bacteria might be able to counter anti-CRISPR defenses through silencing expression of anti-CRISPR genes or targeting anti-CRISPR proteins for proteolysis.
This study lays the groundworks for upcoming discoveries: the author, in fact, do not speculate whether this enzyme could have a key role. Their use could be particularly important as a backup safety measure for gene drive and gene therapy technologies. Anti-CRISPR proteins could also be used to restrict editing activity to particular tissues. The uncertainty of researchers concerning the functions and effects of anti-CRISPR proteins makes it impossible to predict how they might ultimately influence aspects of bacterial physiology that are crucial to human health, such as the spread of antibiotic resistance and toxin genes [8].
References
- Sungwon Hwang and Karen L. Maxwell The CRISPR Journal Volume 2, Number 1, 2019
- Knott, G. J. et al. Nature Structural & Molecular Biology 26, 315–321 (2019).
- Shravanti K. Suresh et al. Nature Structural & Molecular Biology 26, 250–251 (2019).
- Shravanti K. Suresh et al. Nature Structural & Molecular Biology 26, 250–251 (2019).
- (Dong, L. et al. Nat. Struct. Mol. Biol. https://doi.org/10.1038/ s41594-019-0206-1 (2019))
- Stella, S. et al. Cell 175, 1856–1871.e21 (2018).
- Pawluk A, et al Nat Microbiol 1:16085. (2016).
- April Pawluk et al. Nature Reviews Microbiologyvolume16, pages12–17 (2018)